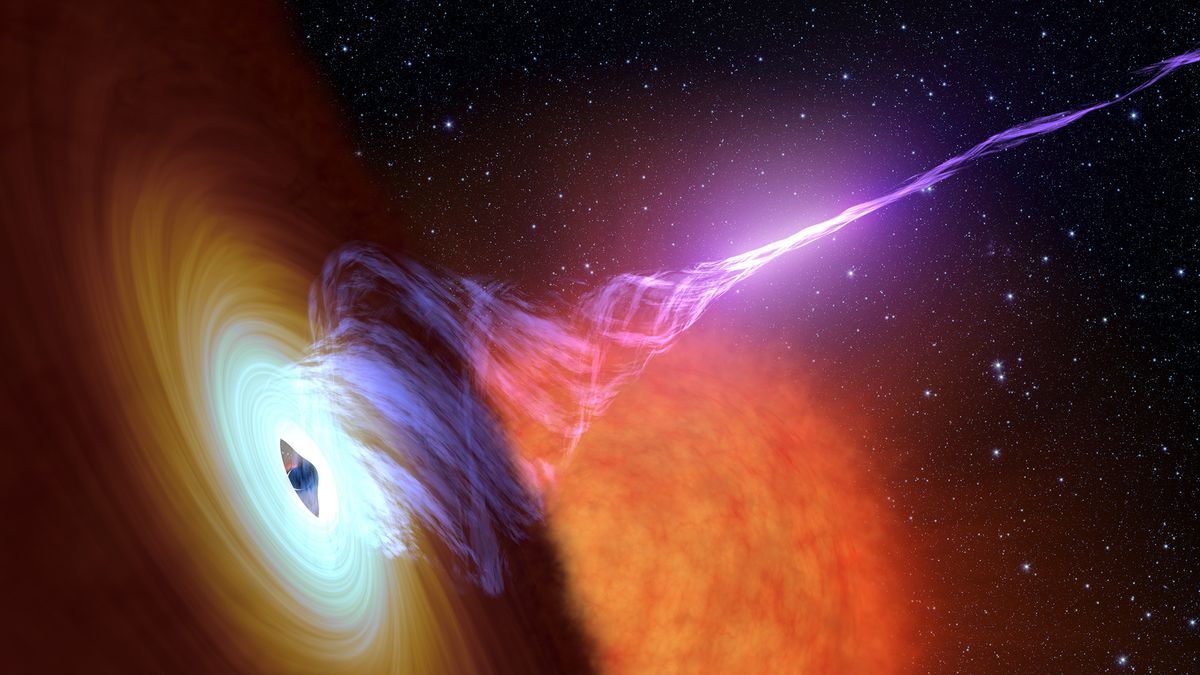
An experiment using beams of protons to probe how plasma and magnetic fields interact may have just solved the mystery of how quasars and other active supermassive black holes unleash their relativistic jets.
Let’s picture the scene at the heart of a quasar. A supermassive black hole, perhaps hundreds of millions — or even billions — of times the mass of our sun, is ravenously devouring matter that is streaming into its maw from a spiraling, ultra-hot disk. That charged matter is called plasma, and it gets gravitationally drawn into the black hole’s surroundings — however, not all of the plasma, which is made from ionized, or electrified, atoms shorn of electrons, is swallowed by the black hole. Indeed, the black hole bites off more than it can chew, and some of the plasma is spat out in jets collimated by the black hole’s powerful magnetic field before that plasma gets anywhere near the event horizon, which is basically the point of no return.
These jets can stretch thousands of light-years into space. Yet, explaining the physics that takes place at the base of the jet, where they’re formed, has eluded scientists.
The answer may have come from researchers at the Princeton Plasma Physics Laboratory (PPPL) in New Jersey, who were able to devise a modification to a plasma-measuring technique called proton radiography.
In their experiment, the researchers first created a high-energy density plasma by firing a pulsed, 20-joule laser beam at a plastic target. Then, they used powerful lasers to instigate nuclear fusion in a fuel capsule filled with deuterium and helium-3. The fusion reactions released bursts of protons and X-rays.
Related: Early galaxies weren’t mystifyingly massive after all, James Webb Space Telescope finds
These protons and X-rays then passed through a nickel mesh filled with tiny holes. Think of the mesh as like a colander for straining pasta; it strains the protons into many discrete beams that then can measure how the expanding plasma plume interacts with a background magnetic field. Because the protons are charged, they’ll follow the magnetic field lines as they are buffeted by the plasma. The X-ray burst acts as a check — because the X-rays pass cleanly through the mesh and the magnetic field, they provide an undistorted image of the plasma to compare to the proton beam measurements.
“Our experiment was unique because we could directly see the magnetic field changing over time,” said Will Fox, the experiment’s principal investigator, in a statement. “We could directly observe how the field gets pushed out and responds to the plasma in a type of tug-of-war.”
They observed in detail the magnetic field bending outward under pressure from the expanding plasma, with the plasma sloshing against the magnetic field lines. This bubbling and frothing of the plasma is known as magneto-Rayleigh Taylor instability, and it created shapes in the magnetic field that look like whirls and mushrooms. Crucially, as the plasma energy decreased, the magnetic field lines were able to snap back. This compressed the plasma into a straight, narrow column not unlike a quasar’s relativistic jet.
“When we did the experiment and analyzed the data, we discovered we had something big,” said PPPL’s Sophia Malko. “Observing magneto-Rayleigh Taylor instabilities arising from the interaction of plasma and magnetic fields had long been thought to occur but had never been directly observed until now. This observation helps confirm that this instability occurs when expanding plasma meets magnetic fields.”
The experiment strongly indicates that quasar jets can thank this sort of reaction of magnetic fields to the expanding plasma for their creation. If the results are a snapshot of what happens around active black holes, that would mean, in the black hole’s accretion disk, conditions become so intense that the plasma in the disk is able to push against the tightly packed magnetic field lines, which can then snap back and push the plasma into a narrow column, almost squirting it away from the black hole. If true, this might be a huge missing piece in our picture of how active black holes operate.
Related Stories:
“Now that we have measured these instabilities very accurately, we have the information we need to improve our models and potentially simulate and understand astrophysical jets to a high degree than before,” said Malko. “It’s interesting that humans can make something in a laboratory that usually exists in space.”
The findings were published on June 27 in the journal Physical Review Research.